AiMM will not be running in 2017.
Growing list of available projects for 2016
List of AiMM faculty
Modeling of optical spectra of molecules and materials for electro-optics. Mentor: Dr. Christine Isborn (Chemistry)
HIV inhibitors formulated into silk materials for prevention of HIV in resource-poor settings. Mentor: Dr. Patti LiWang (Chemistry)
Investigation of protein metamorphosis. Mentor: Dr. Andy LiWang (Chemistry)
Nanomechanical systems in the quantum limit. Mentor: Dr. Lin Tian (Physics)
Polarization of Raman scattering in CdSe quantum dots. Mentor: Dr. Anne Myers-Kelley (Chemistry)
The synthesis of diarylcyclooctatetraenes to study the relationship between their structure and light-absorbing properties. Mentor: Dr. Ben Stokes (Chemistry)
Stiffness control in silk electrospun vascular grafts. Mentor: Dr. Kara McCloskey (BioEngineering)
Swimmers in chaotic flows. Mentor: Dr. Kevin Mitchell (Physics)
Project Descriptions
Modeling of optical spectra of molecules and materials for electro-optics.
Dr. Christine Isborn (Chemistry)
Organic chromophores with interesting optical properties are often embedded in a thin film for materials applications such as electronic semiconductors and optical coatings. Much time and energy has been spent optimizing organic chromophores at the single molecule level for use in solar cells, optoelectronics, optical data processing, and telecommunications. However, these molecular improvements do not necessarily translate into the same significant progress at the macroscopic level. For charge transfer chromophores with donor and acceptor groups, the dipole coupling between chromophores in a thin-film may be so strong that they pack in an anti-parallel fashion that washes out most of the optical properties of interest, for example the molecular nonlinear optical hyperpolarizability β yields the electo-optic effects necessary for optical signaling. Chromophores can have large changes in their absorption spectra and optical properties going from dilute solution to thin-film environments.
This project will involve using electronic structure quantum chemistry methods (such as Hartree-Fock and density functional theory) to model the electrostatics, charges, electronic excitations, and hyperpolarizabilities of nonlinear optical chromophores in solution phase and thin-film environments. Since real materials involve the properties of many molecules in bulk (chromophores coupled together in solvent or in a polymer), we must go beyond the single molecule picture in our understanding of these optical properties. This project will involve taking into account surrounding solvent and the coupling of multiple chromophores to understand how chromophore packing affects the resulting optical properties and shifts them from the results of a single molecule in vacuum. We will also examine which theoretical methods produce results in best agreement with experiment, using UV-Vis absorption spectra as our benchmark data. Necessary background: Physical Chemistry. Computing (unix, command line, Gaussian) experience is a plus.
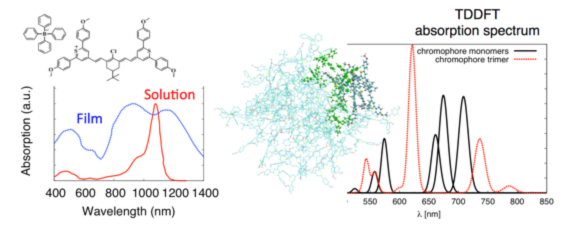
HIV inhibitors formulated into silk materials for prevention of HIV in resource-poor settings.
Dr. Patti LiWang (Chemistry)
We are working with protein HIV inhibitors that have been formulated into easily dissolving silk films. These materials are proposed to be used for the prevention of the sexual spread of HIV. The goal is to obtain materials that maintain stability without refrigeration even at tropical or sub-saharan temperatures and that can mediate the release over time of an inhibitor so that it can provide protection for a week or more to a person at risk for HIV infection. An REU student would obtain experience in producing anti-HIV proteins (and variants) as well as determining the properties of the formulated silk inhibitors. Necessary Background: Organic chemistry (required); Biochemistry (preferred); Excellent grades or very high class standing.
Investigation of protein metamorphosis.
Dr. Andy LiWang (Chemistry)
A rare class of proteins, known as metamorphic proteins, reversibly switch between two distinctly different topological folds, allowing the encoding of two different functions within a single polypeptide chain. The goal of this project is to investigate the metamorphic properties of the clock protein, KaiB. It helps set the pace of a biological clock by slowly metamorphosing between active and inactive states. Necessary Background: Introductory biology with lab, general chemistry, with lab. The student should have learned how to make buffers, know sterile techniques, and other fundamental techniques.
Nanomechanical systems in the quantum limit.
Dr. Lin Tian (Physics)
In this project, the REU student will study effective generation of quantum entanglement between two mechanical modes both coupling to a common superconducting qubit, which is a natural extension of our previous projects1,2 and is a key component for demonstrating the quantum nature of the mechanical modes. Necessary Background: At least one semester of upper-division quantum mechanics (with a grade of B or better).
1. Tian, L. Phys. Rev. B. 2005, 72, 195411.
2. Jacobs, K., Tian, L., Finns, J. Phys. Rev. Lett. 2009, 102, 057208.
Polarization of Raman scattering in CdSe quantum dots.
Anne Myers-Kelley (Chemistry)
A quantum dot is a small, roughly spherical chunk of a bulk semiconductor typically 2 to 8 nm in diameter. In analogy to the quantum mechanical particle-in-a-box problem, making the quantum dot smaller causes its electronic energy levels to become more widely spaced, changing the wavelength of the light that it can absorb or emit. The size tunability of the wavelength, the spectral sharpness of the emission, and the resistance to photochemical damage make semiconductor quantum dots promising materials for solar energy conversion, artificial lighting, and bio-labels for optical microscopy. While the useful optical and electrical properties of semiconductor quantum dots are largely properties of their electronic states, the vibrational modes of the atoms (called phonons in crystalline solids) also influence these properties. Coupling between the excited electrons and phonons is the principal pathway whereby excess energy is dissipated into heat. For most applications this is an energy-wasting pathway, so we would like to minimize it. In order to decide what structures should minimize electron-phonon coupling, we first need to understand the physical parameters of the quantum dot that determine it.
Resonance Raman spectroscopy, a type of inelastic laser light scattering, is the most direct method of measuring electron-phonon coupling in quantum dots and our group has already done considerable work in this area. A further experiment that should be enlightening is a measurement of the Raman depolarization ratio (the extent to which the Raman scattered light is polarized parallel or perpendicular to the exciting laser light) for the CdSe phonons as a function of excitation wavelength. This measures the extent to which the CdSe quantum dots are isotropic (properties the same in all three directions, as expected for a sphere) with regard to both its electronic states and its phonon modes, and will help us develop a correct theoretical model for the electronic excitations and the phonon modes, and the nature of their coupling. The proposed work will involve synthesizing CdSe quantum dots via well developed methods currently in use by our group, learning how to use our microscope-based laser Raman spectrometer, learning the basic theory of Raman depolarization ratios and how they are measured, carrying out calibration measurements on simple liquids, and measuring Raman depolarization ratios on CdSe quantum dots and comparing them to expectations from different theoretical models. Depending on the student’s interests, the project may also include computational simulations of the geometric structures and phonon modes of CdSe quantum dots. Necessary background: first-year chemistry and physics with laboratory; introductory quantum mechanics in either physics or chemistry.
The synthesis of diarylcyclooctatetraenes to study the relationship between their structure and light-absorbing properties.
Dr. Ben Stokes (Chemistry)
Cyclooctatetraene (COT) is a commercially-available, nonaromatic annulene. Its standard ‘flat’ two-dimensional depiction (1, Figure 1A) suggests a planar structure, but in the solid state, it exists in a non-planar tub-shaped conformation with C=C–C bond angles of 126.1° and C=C–H angles of 117.6° (Figure 1A).1 Axially-symmetric diaryl cyclooctatetraene analogs like dibenzocyclooctatetraene (2, Figure 1B) are important ligands for transition metals.2,3 Diaryl cyclooctatetraene analogs even occur in nature, as is the case with the algal alkaloid natural product caulerpin (3).4 Caulerpin is a red pigment that was first isolated in 1970,5 and is found in various tropical and subtropical edible seaweeds of the genus Caulerpa6 and red algae of the genus Chondria.7 It is the main secondary metabolite of Caulerpa racemosa, constituting 2% of the dry weight of the plant. Since an efficient total synthesis of caulerpin has not been reported to date,8 its photophysical properties are not well-understood. Thus, my lab is interested in developing an efficient and modular synthesis of this natural product that will also enable the preparation of analogs. This library of compounds will then be used conduct a systematic study of the structural properties responsible for the molecule’s ability to absorb light. One prospective approach that we will take towards synthesizing caulerpin and its analogs is outlined in Figure 1C. This approach features three consecutive transition metal-catalyzed steps that are highly functional group tolerant, which will allow us to prepare a sizeable library of compounds. Necessary Background: Successful completion of introductory undergraduate organic chemistry lecture and laboratory courses. Students interested in pursuing graduate studies in organic chemistry are excellent candidates for this position.
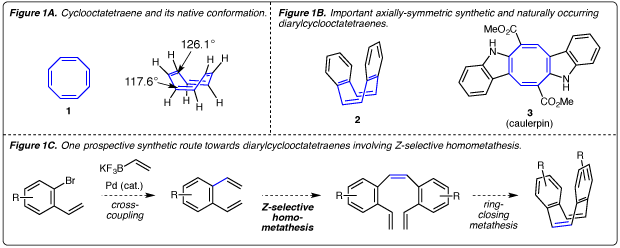
- Kaufman, H. S., Fankuchen, I., Mark, H. Nature 1948, 161, 165.
- Singh, A.; Sharp, P. R. Organometallics 2005, 25, 678.
- Spiess, S.; Welter, C.; Franck, G.; Taquet, J.-P.; Helmchen, G. Angew. Chem. Int. Ed. 2008, 47, 7652.
- Parvez, M.; Ara, J.; Sultana, V.; Qasim, R.; Ahmad, V. U. Acta Crystallographica Section C 2000, 56, e96.
- Aguilar-Santos, G. J. Chem. Soc. C: Organic 1970, 842.
- Capon, R. J.; Ghisalberti, E. L.; Jefferies, P. R. Phytochemistry 1983, 22, 1465.
- Govenkar, M. B.; Wahidulla, S. Phytochemistry 2000, 54, 979.
- Maiti, B. C., Thomson, R. H., Mahendran, M. J. Chem. Res., Synop. 1978, 126.
Stiffness control in silk electrospun vascular grafts.
Dr. Kara McCloskey (BioEngineering)
Our goal in this project will be to control stiffness of electrospin silk tubes for developing vascular grafts that can mimic stiffness native vessels. The project will proceed in two phases, and the REU researcher will have the assistance of a graduate student throughout. First, we will train to generate silk solutions and electrospin the tubes, varying a number of parameters that will lead to variable nanofiber sizes, correlating with material stiffness. The student, with the help of the graduate student mentor, will then measure the stiffness of the silk material using atomic force microscopy. Necessary Background: Junior or higher standing is preferred.
Swimmers in chaotic flows.
Dr. Kevin Mitchell (Physics)
Recently, there has been significant research interest in the influence of fluid flow on swimming organisms, with potential applications to schools of fish in the ocean, swarms of autonomous underwater vehicles (AUVs), or microorganisms in micro-fluidic environments. The swimmer dynamics is particularly rich when the underlying fluid flow generates chaotic mixing. This project will take a theoretical dynamical-systems approach to studying how a swimming organism explores its fluid environment. Important questions include: what regions in a fluid are accessible to a swimming organism and what kinds of dynamical structures are responsible for restricting access? This project will build on initial results from our group showing that certain dynamically defined curves, called swimming invariant manifolds, or SwIMs, are at least partly responsible for confining swimmers to particular regions of a fluid. Necessary Background: Some experience solving ODEs and programming. Completion of an upper-division classical dynamics course and some experience programming in MatLab are preferred.
Additional AiMM Faculty
- Dr. Jay Sharping (Physics)
- Dr. Hrant Hratchian (Chemistry)
- Dr. Erik Menke (Chemistry)
- Dr. Jing Xu (Physics/Biophysics)
- Dr. Tao Ye (Chemistry)
- Dr. Roland Winston (Physics/Engineering)
- Dr. Linda Hirst (Physics)
- Dr. Sai Ghosh (Physics)
- Dr. Ajay Gopinathan (Physics)
- Dr. Alexander Noy (Chemistry)
- Dr. Ryan Baxter (Chemistry)
- Dr. Michael Scheibner (Chemistry)
- Dr. Anand Subramaniam (Engineering)